I hope by now you’ve read my article, Scale. It hints at something odd about the Universe.
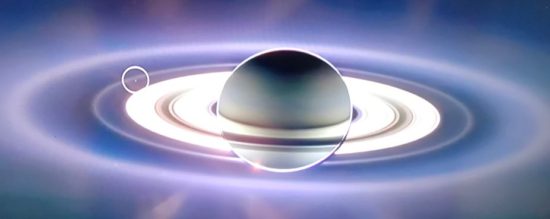
When looking up into the night sky people sense the vast distances between the objects they see. But when looking down at the ground they experience something different. It seems that objects are solid, without internal structure.
No one can know by looking that solid objects are made of tiny molecules separated from each other by tiny gaps. Even sophisticated instruments like microscopes provide experimenters with no chance of seeing any molecules. Molecules are too small.
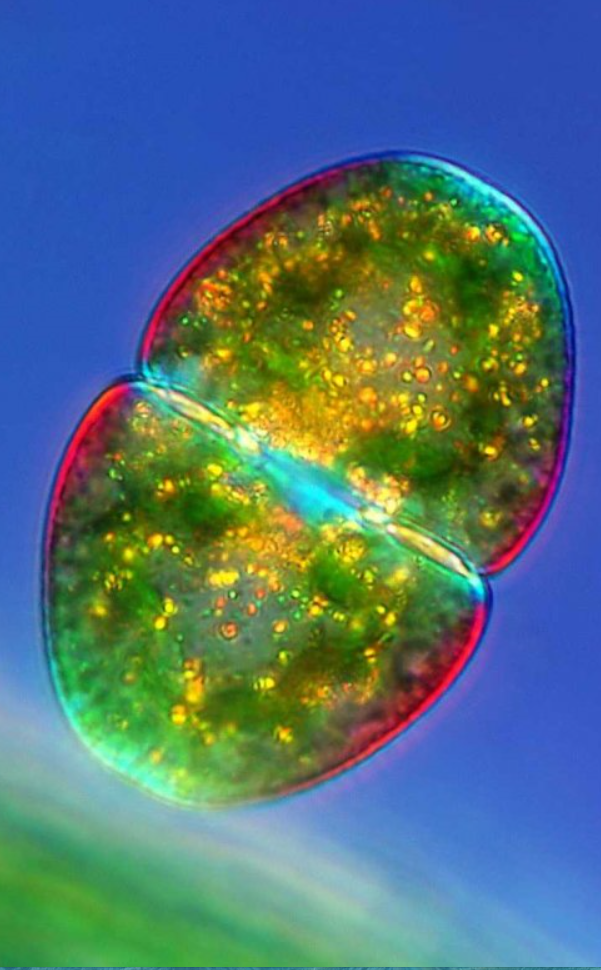
Think about it. No one has ever seen a molecule.
No one.
Computers have created pictures based on programming rules and data from sensors to provide an idea of what molecules might look like — if molecules lived in the world at human scales and reacted to sensors and probes the way people do. But, of course, they don’t.

Few professors emphasize to kids in freshman chemistry, as far as I know, that they are learning the rules from models of molecules which have been invented — fabricated — to help make sense of lab experiments done on substances that are able to be touched by hands and seen with unaided eyes.
Worse, visual models can never be realistic when applied to the objects scientists call atoms. Atoms are what molecules are made from. They must be completely fanciful. It’s true. Scanning tunneling microscopes (STMs) have been used since 1981 to “feel” the forces of atoms with “nano” probes. Based on plots of these forces, pictures of atoms that look like stacked billiard balls are generated by computer algorithms.
Whatever it is that atoms are, they aren’t resolvable with light, which is what brains use to view and imagine things. The constituents of atoms are quantum objects that don’t behave like anything familiar to ordinary life. Everything folks think they know about atoms is made-up by scientists who are struggling to make sense of the way substances behave under every set of experimental circumstances imaginable.

Scientists have invented models of atoms, which are made from protons, neutrons and electrons (that whirl inside s, p, d, f & g orbitals) — whatever — to aid their thinking. No one examines an atom to see if it looks like its model, because they can’t.
Whatever it is scientists are modeling can’t be seen by eyes or microscopes. If the model helps scientists predict what will happen in experiments, they are OK with it. Physicist Stephen Hawking calls it model-dependent realism. The models are good enough.
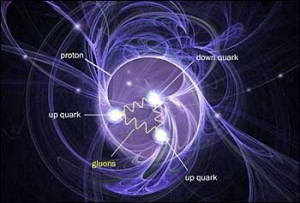
During the past fifty years or so experiments have revealed new layers of complexity, which older models of the atom don’t address. So scientists have devised new models to help them reason more clearly about the strange events they were observing.
Scientists invented more structures and more “particles” — quarks being the best known — to explain and simplify the fantastic results of recent experiments.
Before the idea of the quark, scientists struggled with the complexity of a theory that included hundreds of particles. Frustrated physicists referred to the complexity as the “particle zoo.” After the theory of quarks was accepted, the number of particles in the “standard model” dropped to seventeen.
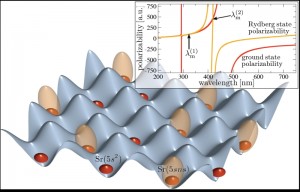
Some current models of the subatomic world postulate point-size masses immersed in vast volumes of interstitial space. These models reflect the mathematics used to build them, but are probably not helpful for understanding what is really going on.
John Wheeler, the theoretical physicist who coined the terms worm-hole and quantum-foam, said this about the very small: …every item of the physical world has at bottom — a very deep bottom, in most instances — an immaterial source and explanation…
At the smallest scale anyone can realistically work with — the scale of molecules — the structure of matter is dense. The space between molecules in a lattice is not much larger than the size of the molecules.
The force fields inside the molecular lattice are powerful — powerful enough to make the lattice impermeable. Vast volumes of empty space don’t exist within. Matter and energy seem to be working together in a kind of soup of symbiotic equivalence.
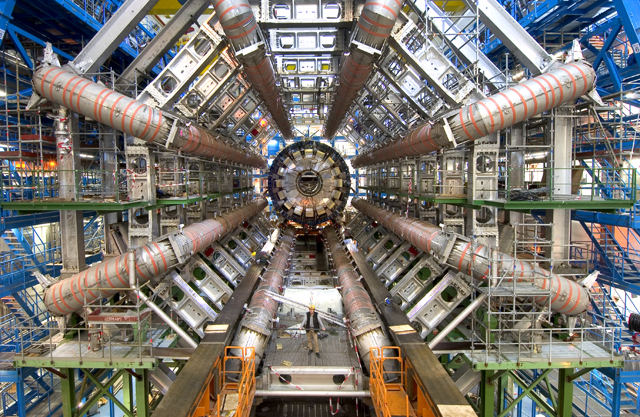
It might be reasonable to expect that at smaller scales, forces and fields take over. Matter, as folks usually think of it, is gone. Fields (whatever they might really be) predominate. When fields interact with detectors, the detectors provide data as if they interacted with massive particles immersed in vast volumes of empty space.
It might be an illusion that leads people to miss an underlying reality of smaller scales — descent into the abyss of small scales reveals regions of disproportionately less space, not more. The stairway to smaller scales may lead to densities of force/energy and limitations of space/time like those found in black holes.
In a typical black hole — a hundred million may inhabit the Milky Way Galaxy — a typical event horizon might have a circumference of thirty miles. Its diameter could measure millions of miles. Dimensions like these violate the Euclidean rules of geometry everyone expects. According to the rules, a spheroidal event horizon with a thirty mile circumference can’t measure more than ten miles across.
A diameter of millions of miles for an object with a thirty mile circumference seems crazy at first, until the implications of relativity are examined, which demand that the volume of space and span of time within a black hole be densely distorted and wildly warped.
A black hole contains within its volume the energy-equivalent of all the matter of the collapsed and vanished star that formed it plus all the energy-equivalent of any other matter that may have fallen into it. It is a region mostly devoid of matter — it is energy rich but matter impoverished — analogous perhaps to those tiny spaces some think might exist within and between atoms and inside the sub-atomic realms of ordinary matter.
Said plainly, whatever exists at tiny scales is not understood, but maybe knowledge about black holes can provide insights. I think so. The problem: knowledge about black holes is speculation based on mathematics; unless we are already living inside a black hole, no one can experimentally verify the ideas of smart and talented people like Stephen Hawking, for example.
The problem of understanding the very small is serious. The most advanced particle detector humans can afford to build blows up protons to examine their debris field. The detector “looks at” debris that measures about 1/100th the size of the protons it smashes. Accelerators — like the one at CERN — can’t “see” anything smaller.
From these tiny pieces of accelerator-trash theories of nature are fashioned. The inability to resolve the super small stuff is a problem. No one can see quarks, for example. Scientists at the ALICE Lab at CERN hope to fashion a “work around” by using the nuclei of iron atoms to make progress in the coming years.
To examine debris at Planck scales — which would answer everyone’s questions — requires a resolution many trillions of times greater than CERN can deliver. Such a machine would have to be much larger than the one at CERN. It would have to be larger than the solar system. In fact, it would have to be larger than the Milky Way Galaxy. Even then, the uncertainty principle guarantees that such a machine could not remove all the quantum fuzziness from whatever images it might create.

According to IAS theoretical physicist, Nima Arkani-Hamed, it might be possible to burrow down to an understanding of the very small by using pure thought — as long as it is consistent with the mathematics that is already known for sure about quantum physics and relativity theory. The problem is, no one will ever be able to confirm the new models by doing an experiment.
The good news, Nema says, is that constraints imposed by knowledge already confirmed may so reduce the number of paths to truth that somebody might find a way that is unique, sufficient, and exclusive. If so, folks can have confidence in it, though experimental verification may lie well beyond the reach of technology.
But again, fundamental problems — like trying to observe an intact, whole atom — remain. No technology of any kind exists that will permit anyone to observe an entire atom at once and resolve its parts.
Physicists are reduced to using what they learn from observing atomic-scale debris to help fashion, in their imaginations, what such an entity might “look” like. No one will ever have the holistic satisfaction of holding an atom in their experimental hands, observing it, and pushing on its quantum-endowed components to see what happens.
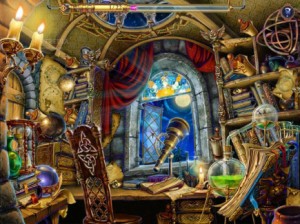
Where does it all lead? At this stage in its history, science is struggling to figure out what’s happening.
In the USA, (where the big money is) science seems to serve the military and companies struggling to create products that capture the imagination and pocketbooks of a buying public. For the moment at least, science is preoccupied with serving better those who pay for its services.
But someday — hopefully soon — scientists may refocus their considerable talents on the questions that really matter most to people:
Where are we? What, exactly, is this place? Is anyone in charge?
Billy Lee